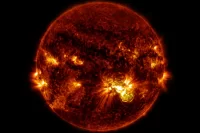
What do you get when a Bates biology professor joins a paleobotanist, hydrologist, plant physiologist, and plant anatomist in a research project?
You get the answer to a question that has long vexed scientists: How did ancient aquatic plants adapt to dry-land living?
Co-authored by Professor of Biology Brett Huggett, a paper published recently in the prestigious journal Science explains how one physiological adaptation sparked a major evolutionary shift in plant life on Earth.
Hundreds of millions of years ago, the earliest land plants developed an internal plumbing system — roots and vascular conduits made of xylem — needed to pull water from soil and then distribute it, along with nutrients, throughout their bodies.
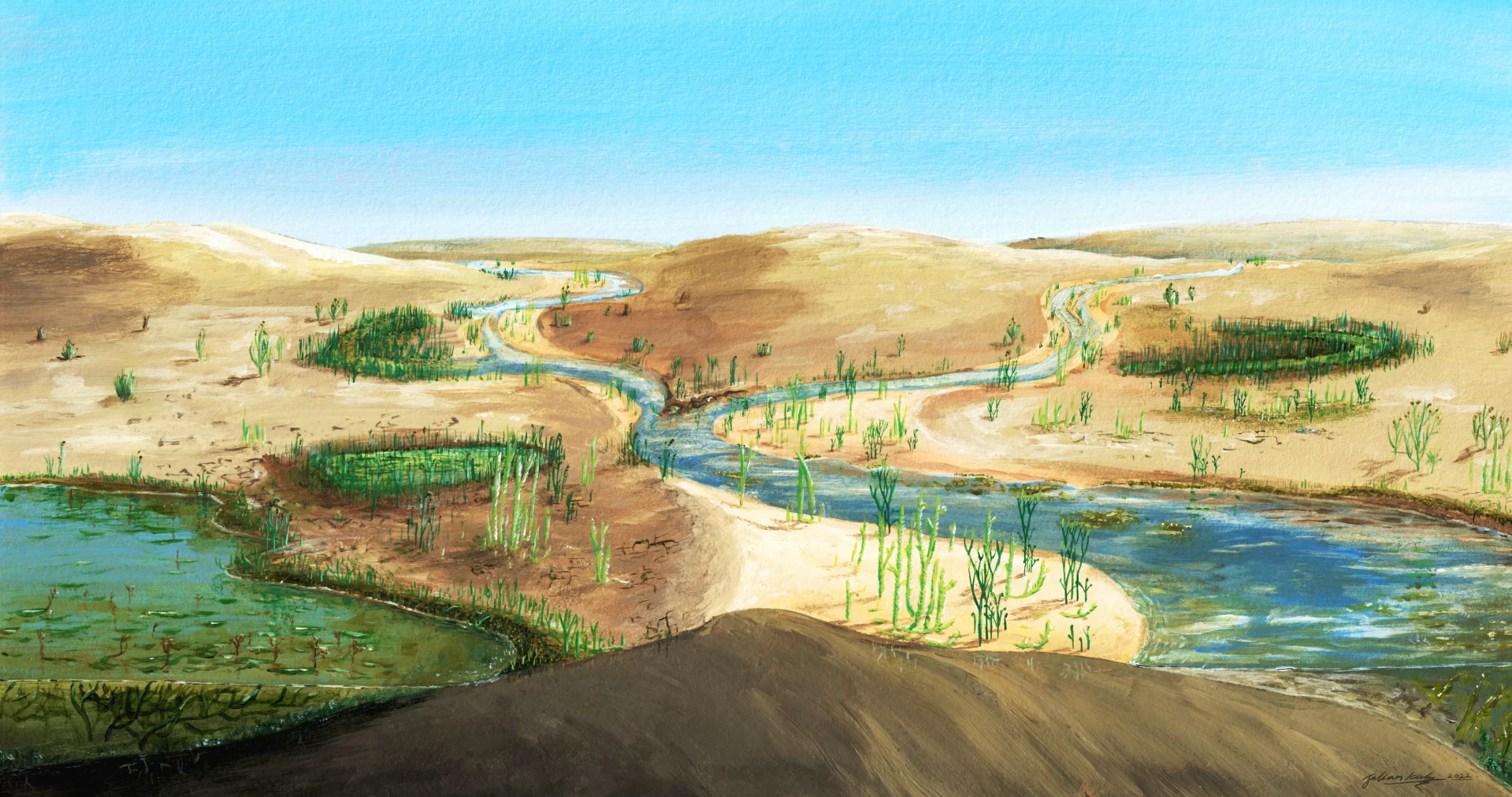
Still highly vulnerable to drought, these plants, just a few inches tall, had to stick close to water: on the shore, on river banks, and in swamps. Then around 400 million years ago, something happened that allowed their expansion to much drier habitats. “It was a major transition,” says Huggett. “From being completely restricted to wet habitats to being able to colonize drier environments.”
For a century, scientists have known that as plants expanded to dry land, their vascular network of xylem conduits evolved and became more complex. But scientists could never “pinpoint an environmental driver for why that happened,” says Huggett, whose research was supported by a competitive Phillips Fellowship from Bates.
Left with assumptions, scientists leaned on coincidence, figuring that as plants got taller, “they became more complex in their growth form: more branches, more leaves, and in turn more complex in their vascular systems.”
But it was no coincidence, as Huggett and his colleagues report in the paper, whose lead author is Martin Bouda of the Institute of Botany of the Czech Academy of Sciences. In fact, plants developed their complex vascular network because of evolutionary pressure, from drought, which in turn allowed them to live and thrive on dry land.
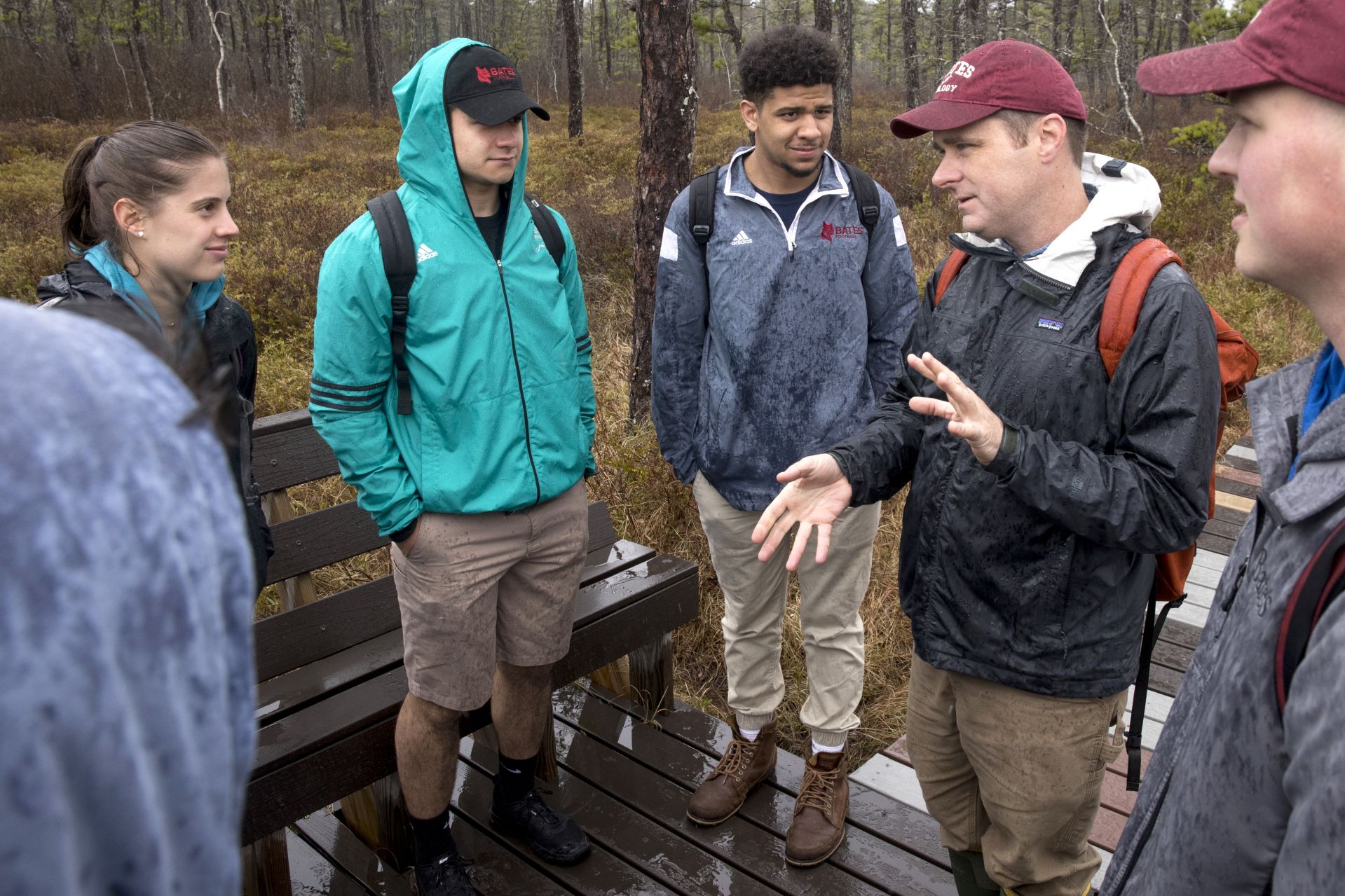
Huggett’s expertise is specifically “how plant vascular systems function under drought,” he explains. To understand that, here’s a primer on how water moves through a plant.
In humans, the heart pushes blood into arteries and then to the far reaches of the body. That’s positive pressure. In a plant, as water exits the plant through its leaves, more water is pulled from the ground through its roots. That’s negative pressure, like a siphon. “Imagine sucking a soda through a straw,” says Huggett.
When a plant dries out during drought, their xylem conduits can develop an air bubble, a blockage — an embolism — that can be just as damaging as an embolism in a human artery. The conduit, which is “essentially a hydraulic rope, snaps. It becomes blocked and rendered dysfunctional.” This is known as hydraulic failure.
If drought conditions intensify, “air bubbles can spread from one conduit to the next, and it can become systemic and deadly to the plant,” he says. (There are other ways a plant or tree’s xylem network can be damaged, “like when you see a woodpecker hammering away on a tree, they’re puncturing holes into the xylem network,” says Huggett, who maintains, with his students, the Canopy, a comprehensive resource about campus trees.)
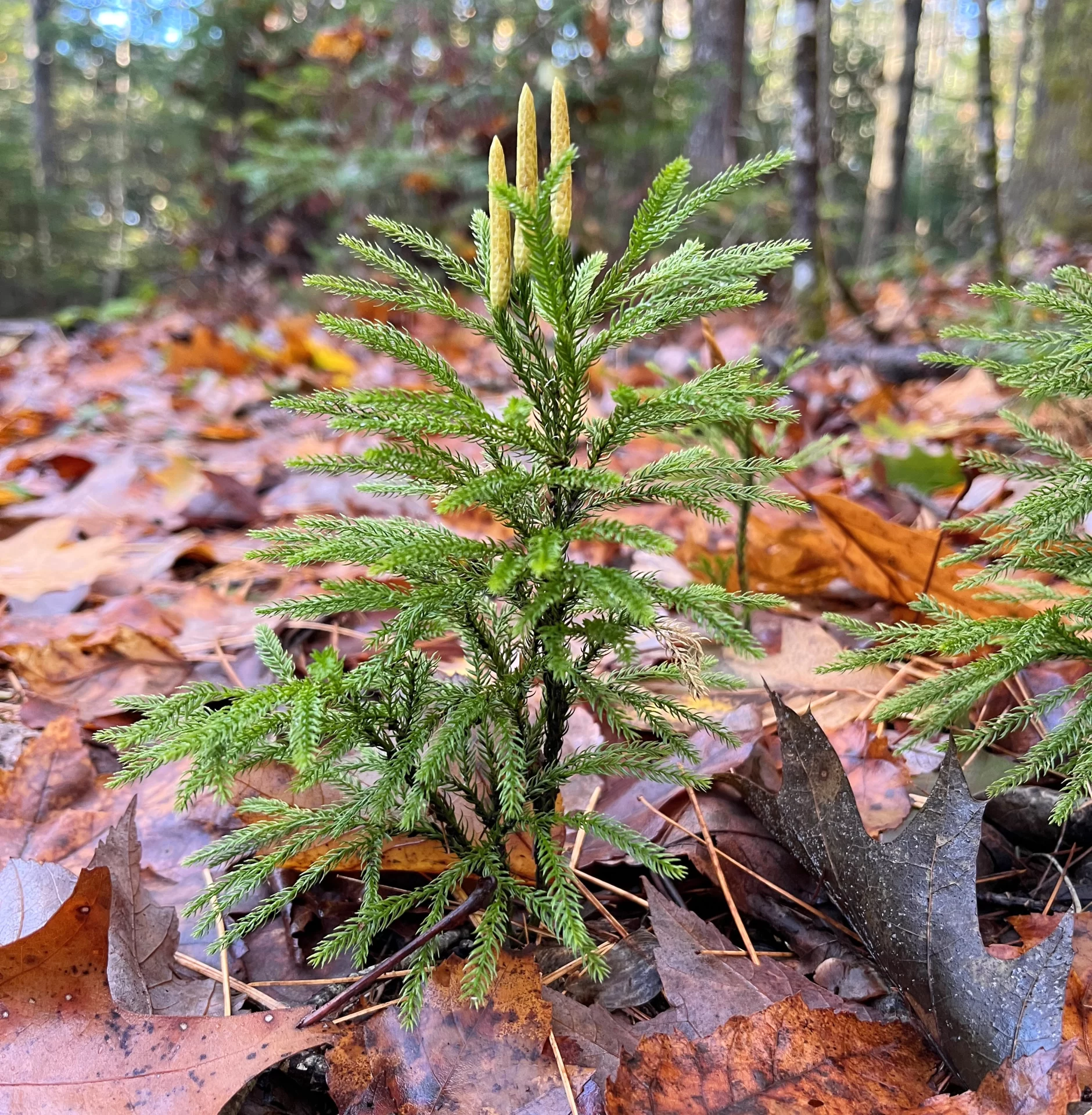
In early land plants, specifically seedless vascular plants, the xylem strands were tightly grouped in a cylinder, like a bunch of straws bound by a rubber band. “The conduits were packed right up against one another,” Huggett says. “If you got an air bubble in one conduit, it can then go to several more. Under increasing drought pressure, the embolism can spread exponentially.”
But over time, the plants’ cylindrical strands became “more bifurcated and spread out, and in some species, star shaped,” he explains. This change was helpful: an air bubble couldn’t easily spread systemically because “there’s a restricted amount of conduits adjacent to one another.” In other words, the plants had protection from hydraulic failure during drought.
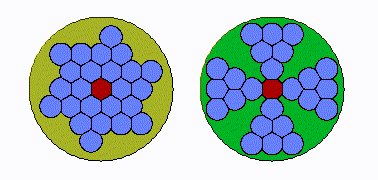
To test whether this change was driven by evolutionary pressure, the researchers used their expansive understanding of plant life on earth, from hundreds of millions of years ago to today, to examine the anatomy of both extinct land plants and their living relatives.
Huggett and his fellow researchers found that changes had to be driven by evolutionary pressure — specifically a plant trying to protect itself from drought.
Using microscopy and anatomical analyses of fossils and living plants, tied with measurements of drought resistance in living plants, Huggett and his fellow researchers found that changes in the arrangement, size, and complexity of xylem strands had to be driven by evolutionary pressure — specifically a plant trying to protect itself from drought.
“Any change in the vascular architecture from that ancestral cylindrical form — any change in getting more complex — led to greater ability to avoid the negative impacts of drought,” says Huggett. With that protection came the ability to live and thrive in dry ground, the previously unknown environmental driver that “allowed plants to then colonize even drier and drier habitats.”
In addition to Huggett and Bouda, the paper’s authors are Craig Brodersen and Kyra Prats of the Yale School of the Environment; Jay Wason of the School of Forest Resources, University of Maine; and Jonathan Wilson of the Department of Environmental Studies, Haverford College.